|

COMPUTER
simulation is changing the nature of scientific discovery by becoming
a full partner with theory and experiment. Nowhere is that transformation
more visible than at Lawrence Livermore, where scientists are relying
increasingly on computer simulations, especially those based on
high-resolution, three-dimensional models. Such simulations, however,
require the enormous computational horsepower of the latest generation
of supercomputers.
To ensure that all Livermore
programs and researchers have the possibility of accessing high-performance
computers, the Laboratory created the Multiprogrammatic and Institutional
Computing (M&IC) Initiative in 1996. The initiative has grown
substantially in the last few years and currently serves more than
a thousand users, including outside collaborators, with some of
the most powerful computers available. Indeed, virtually every Livermore
unclassified programfrom atmospheric sciences to biosciencebenefits
from the computing resources provided by the initiative.
M&IC is a partnership
between the Laboratory and its research programs to bring high-performance
computing to every researcher, says Mike McCoy, head of Livermores
Scientific Computing and Communications Department. M&IC recognizes
that no matter what their mission, scientists should have ample
access to centralized, high-performance computers far more capable
than the computational resources that individual departments could
afford to purchase and support. M&IC leaders, under the direction
of McCoy and former Computation Associate Director Dave Cooper,
have worked with key researchers around the Laboratory to create
a centralized operation that helps all researchers do better science
through more advanced simulations.
McCoy notes that his departments
goal is to make the discovery environment that is evolving
in the Accelerated Strategic Computing Initiative (ASCI) available
to all researchers. ASCI is an element
of the National Nuclear Security Administrations Stockpile
Stewardship Program to assure the safety and reliability of the
nations nuclear weapons in the absence of underground testing.
(See S&TR, June 2000, pp. 414.) In the ASCI computing
approach, scientists are supported by powerful simulation tools
that make possible both the generation of raw scientific data and
the manipulation of this information to enhance understanding.
The institutional nature
of the initiative makes it possible for anyone with a good idea
to perform teraops-scale (more than 1 trillion mathematical operations
per second) parallel computing to achieve breakthrough scientific
discoveries. First developed in the late 1980s, parallel computing
attacks huge mathematical problems with a number of identical (and
typically inexpensive and common) processors simultaneously sharing
a computational task. Parallel computing differs from traditional
supercomputing, in which a few high-cost, specially designed vector
processors perform numerical calculations.
Parallel computing
brings formerly insoluble problems into solution range and makes
formerly difficult-to-solve problems routine, McCoy says.
Examples of parallel supercomputers include the Laboratorys
ASCI supercomputers manufactured by IBMthe classified Blue
Pacific (3.9 teraops) and White (12.3 teraops) and the unclassified
Blue (0.7 teraops) and Frost (1.6 teraops)and M&ICs
TeraCluster2000 supercomputer, built by Compaq, operating at 0.7
teraops.
McCoy explains that when
the initiative was launched in the mid-1990s, many scientists, having
experienced difficulties in securing sufficient and sustained access
to unclassified centralized computing, had forsaken high-performance
computing entirely. Instead, they had taken advantage of the opportunities
presented by new and relatively inexpensive and powerful desktop
computers in their offices or used terminals tied to scientific
workstations owned by their research programs. He calls the situation
at the time a desktop diaspora.
While desktop workstations
continue to be an important tool for scientific computing research,
these machines do not always provide the necessary computational
power. Exclusive recourse to workstations, in the absence
of access to the most powerful centralized systems available, could
have left many Lawrence Livermore researchers unequipped with the
computer tools they needed to remain competitive in the next decade,
says McCoy.
Until this time, most
scientists were performing two-dimensional simulations because three-dimensional
simulation was quite difficult, if not impossible, with the computers
at their disposal, says Greg Tomaschke, M&IC computing
systems leader. Livermore has a long history of preeminence
in computational simulation, and people were concerned theyd
start to lose this advantage. M&IC has ensured that Livermore
researchers are at the forefront of simulation science, he says.
On the Horizon:
The Terascale Simulation Facility
|
Looking
to the future, Lawrence Livermore managers are planning a new
facility to house their newest supercomputers. Called the Terascale
Simulation Facility, the building will cover 25,000 square meters
and have offices for 288 people. A two-story main computer structure
will provide the space, power, and cooling capabilities to simultaneously
support two future supercomputer systems for the National Nuclear
Security Administrations Accelerated Strategic Computing
Initiative (ASCI). Nearby, the Livermore Computing Center will
house the unclassified computing resources of the Multiprogrammatic
and Institutional Computing Initiative.
The computer structure
will be flanked on the south side by a four-story office building.
The building will have space for Lawrence Livermore staff, vendors,
and collaborators from the ASCI University Alliance and visiting
scientists from Los Alamos and Sandia national laboratories.
Lawrence Livermore computer
managers say that no existing computer facility at Livermore
is adequate, nor can any be modified sufficiently, to accommodate
the newest supercomputers, which are the largest computational
platforms ever built. These platforms are so powerfulexceeding
50 teraops, or 50 trillion operations per secondthat they
are increasingly labeled ultracomputers. Current plans are to
site a 60-teraops system in the Terascale Simulation Facility
by 2004. |

The
Terascale Simulation Facility will house the largest supercomputers
ever built and offices for nearly 300 people.
|
Serving
Programs and Individuals
The M&IC is so named
because it serves both research programs (multiprogrammatic) and
individual (institutional) researchers. A research program can either
purchase a block of time on existing machines or, more popularly,
share in the investment in new equipment, which gives the program
access proportional to its investment.
The research program designates
a principal investigator to select users of the resources it has
paid for. The Livermore Computing Center furnishes the principal
investigators with Internet-based records of the computing time
the program is entitled to and how much of the time has been used,
in total or by individual researcher. A hotline is available to
answer technical computing questions and provide account information.
M&IC also grants computer
time to individual researchers, independent of their connection
to a program, whose work is often viewed as less mainstream than
most efforts in their particular research specialties. The researchers
are selected through a short proposal process by the Institutional
Computing Executive Group (ICEG), composed of users from the Laboratorys
research directorates. The ICEG provides general oversight of M&IC,
in cooperation with the Computation associate director and the Laboratorys
deputy director for Science and Technology.
The ICEG is the most
important Livermore Computer Center link into the user community,
says McCoy. The M&IC Initiative is based on the strong
bonds of support and advice that exist between the ICEG and the
center. As a result, Livermore supercomputing has become an institutional
resource much like the library, a place where researchers from any
program can expect resources to support their research.
Researchers can monitor the
status of their simulation from their desktop computer. Those who
sign up for long simulation runs can see their place in the queue.
Once the simulation begins, they can open a window on their computer
screen to watch the calculations. Weve made investments
in customer (user) support that significantly exceed the norm for
computing centers worldwide because we know the extraordinary challenges
scientists face with writing and running large parallel calculations,
says McCoy.
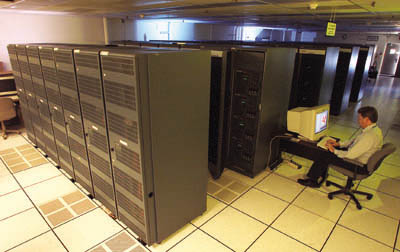
|
The
TeraCluster2000 (TC2K) Compaq supercomputer, operating at 0.7
teraops (trillion operations per second), has 512 central processing
units
arranged in 128 nodes,
256 gigabytes of memory, and 10 terabytes of
disk space. |
Providing
Capacity and Capability
Under the M&IC
Initiative, the Livermore Computing Center has acquired increasingly
more powerful clusters or groups of computers. The computers are
of two conceptually different kinds: capacity computers and capability
computers. Capacity computing is designed to handle jobs that dont
require a lot of computing horsepower or memory. It is often used
for quick turnaround of a large number of small to moderately sized
simulations.
Capability computing uses
a substantial fraction of the entire computing power of a supercomputer
to address a large-scale scientific simulation in three dimensions.
The driver for capability computing usually is the need for
large amounts of memory, which means harnessing many processors
to work together, says Tomaschke. A capability computing resource
can only serve a few users simultaneously.
McCoy
says that any effective computational environment is supported by
a capacity foundation. Capacity allows users to develop the
applications and work the studies that are necessary to conceive
of, develop, and debug capability applications, he says. M&IC
managers, working with the ICEG, developed a strategy early on to
first build a capacity foundation and keep it current. They then
devised a second strategy to build access to capability computing,
either through a partnership with the ASCI program or through unique
research and development relationships with major vendors. Both
strategies have proved effective.
The Compaq 8400 Compass Cluster
was the first capacity computer resource sponsored by the M&IC
Initiative. Delivered in 1996, the Compass Cluster consists of 8
nodes (rack-mounted computers), with each node possessing between
8 and 12 central processing units (CPUs, or microprocessors). In
all, 80 CPUs provide 7 gigaops (7 billion calculations per second)
of computing power, 56 gigabytes of memory, and about 900 gigabytes
of disk space. A replacement for Compass, scheduled to arrive late
this year, will provide a total of 192 gigaops.
To increase capacity computing,
M&IC acquired TeraCluster in late 1998. In all, TeraCluster
consists of 160 CPUs, 80 gigabytes of memory, and over 1.5 terabytes
(trillion bytes) of disk space and provides about 182 gigaops of
computing power. It is closely integrated with the Compass Cluster.
In September 2000, the Livermore
Computing Center took delivery of the Linux Cluster, which generates
42 gigaops of computing power. It is composed of 16 advanced Compaq
nodes, with each node having 2 CPUs and 2 gigabytes of memory. The
machine is used to increase computing capacity and provide users
with an opportunity to evaluate the potential advantages of the
Linux operating system. McCoy says that Linux represents a radical
departure because it is not a proprietary operating system. Success
with the machine could lead to the procurement, next year, of Linux
parallel processing systems using a high-performance interconnect
and advanced Intel processors.
The M&IC Initiative has
also acquired a system manufactured by Sun Microsystems called Sunbert,
which provides 12 gigaops of power with 24 CPUs, 16 gigabytes of
memory, and approximately 600 gigabytes of disk space. The system
is designed to allow access by Livermore researchers who are foreign
nationals from sensitive nations.
 |
TA
sequence of snapshots from a simulation showing how a growing
fracture deforms a stressed copper crystal at the level of atoms.
The first row shows no defects. The second row shows defects
forming on the surface. The third row shows defects shooting
off from the surface in a process that forms a large hole. |
Capability
Power
The M&ICs most
important capability platform, the 680-gigaops TeraCluster2000 (TC2K)
parallel supercomputer, arrived last year. The machine was the result
of a three-year Cooperative Research and Development Agreement between
computer scientists at Livermore and Compaq Computer Corporation
to evaluate a new supercomputer design based on Compaqs 64-bit
Alpha microprocessor and Quadrics Corporations interconnects
and software. The alliance resulted in the Compaq SC Series of supercomputers,
of which TC2K is serial number 1.
Tomaschke says that the most
important aspect of Livermores role in the collaboration with
Compaq was providing advice based on many years of experience with
supercomputers. We gave Compaq important feedback about what
scientists require for doing three-dimensional simulations, such
as operating enormous file systems.
The
TC2K consists of 128 nodes, with 4 Alpha processors per node. In
total, the machine has 512 CPUs, 256 gigabytes of memory, and 10
terabytes of disk space. The 128 nodes are partitioned like a giant
hard disk. The largest partition is dedicated to the most complex
simulations, while a small partition permits a researcher to interact
with the machine in real time. Occasionally, all nodes are freed
up for a single task, such as experiments to determine if a code
will scale properly when the number of nodes increases sharply.
Limited availability of TC2K
began early this year, with 25 projects (involving about 100 researchers)
shaking down the machine. It became generally available
in August, vastly increasing computing capability to all unclassified
researchers.
TC2K represents one of three
capability resources. The second resource is ASCI Blue, the 740-gigaops
unclassified portion of the ASCI Blue Pacific system, which has
282 nodes with 4 IBM Power PC processors each. The third resource
is ASCI Frost, the unclassified version of ASCI White. This system
features 68 nodes with 16 powerful 1.5-gigaops IBM processors each
and 16 gigabytes of total memory. This computer peaks at 1.6 teraops
and is both the most modern and most powerful unclassified computer
on site. Although both Blue and Frost are primarily dedicated to
the ASCI mission, significant access has been made available to
a number of Livermore science teams.
TC2Ks capability, combined
with that of ASCI Blue and ASCI Frost, provides unprecedented unclassified
computing capability for a national laboratory, says McCoy. Researchers
perform code development and limited simulations on capacity machines,
complex three-dimensional simulations on TC2K and Blue, and the
most demanding runs on Frost. Access to the limited unclassified
ASCI resources is extremely competitive.
 |
The
TC2K supercomputer is used to gain insight into the human Ape1
enzyme, a protein that repairs DNA. The simulation at left is
a healthy protein. The simulation at right is a version of the
protein that contains a single amino acid substitution. This
variant shows much more motion in the front loop of the protein;
the motion is a means of recognizing DNA damage. The coloring
indicates the amount of intramolecular motion, with reddish-brown
being the least motion and greenish-blue the most motion. |
Never
Before Attempted
The resources provided by
the M&IC Initiative are permitting researchers to generate simulations
that, in many cases, were never before attempted for lack of computing
power. As a result, the Laboratory is at the forefront of simulating
a wide range
of physical phenomena, including the fundamental properties of materials,
complex environmental processes, biological systems, and the evolution
of stars and galaxies.
For example, physicist Burkhard
Militzer of the Quantum Simulation Group in the Physics and Advanced
Technologies Directorate is using TC2K to simulate how gases such
as hydrogen and oxygen behave under extreme pressure and to compare
those simulations with results of gas-gun experiments done at Livermore.
Militzer uses JEEP, a parallel supercomputer code developed by Livermore
physicist Francois Gygi. The simulations typically require upward
of 300 hours of processing time. Because there is a time limit of
12 continuous hours on TC2K, the simulations run in chunks.
Militzer
says that he would like to use JEEPs quantum mechanics capability
to simulate the weak hydrogen bonds that keep two DNA strands in
their helix. Its very difficult to do accurately because
of all the water molecules surrounding the DNA, he says. TC2K
enables a new class of projects. I wouldnt even begin to think
about running a simulation of DNA hydrogen bonding without having
TC2K.
 |
The
TC2K supercomputer is able to perform three-dimensional simulations
of seismic wave propagation with Livermores E3D code that
is optimized for parallel supercomputers. The image on the left
used 1 central processing unit (CPU) and 0.3 gigabyte of computer
memory in a traditional acoustic simulation of an underwater
deposit. The image on the right used 240 CPUs running for up
to 18 hours and 85 gigabytes of computer memory to generate
a full-physics elastic simulation. The image contains a great
deal more detail, including seismic S (shear) waves that travel
in the earth. |
Examining
the Birth of Cracks
Physicists Robert Rudd and
Jim Belak run simulations on the Compass Cluster. The simulations
examine in microscopic detail the birth of fractures in metals such
as copper under the extreme stresses of a shock wave. The molecular
dynamics simulations are done in a nanometer-scale box holding about
one million virtual atoms.
The simulations, actually
a sequence of snapshots from a movie, depict a copper crystal that
is deformed by the growing fracture over a period of 60 picoseconds
(trillionths of a second). Only the atoms at the fracture surface
or in crystal defects are shown. The defects, known as dislocations,
can be seen shooting off in a process that forms an increasingly
large hole or fracture. Rudd says, Were interested in
learning more about how voids grow and how the material deforms
around them.
We have great confidence
in our simulations, adds Rudd. He is planning to use ASCI
Blue to vastly expand the length of the simulated piece of metal
and to simulate much longer time periods.
Lawrence Livermore biological
scientists in the Computational Biology Group have been one of the
most visible users of the M&IC Initiative. (See S&TR, April
2001, pp. 411.) The researchers have produced stunning depictions
of DNA and proteins that reveal the exact mechanisms of key biological
processes. Parallel supercomputers are ideal for this kind of simulation
because they excel at modeling the interactions of large numbers
of atoms contained within biological macromolecules.
Researcher Daniel Barsky
of the Computational Biology Group has been studying the dynamics
of Ape1, an enzyme responsible for repairing a common form of DNA
damage called abasic lesions. Barsky uses the TC2K to compare the
degree of intramolecular motion of normal Ape1 with a variant found
in which the enzyme contains a single amino acid substitution.
Computer scientist and geophysicist
Shawn Larsen has been using TC2K to do three-dimensional simulations
of oil exploration problems and seismic wave propagation. Larsens
E3D code is optimized for parallel supercomputers. (See S&TR,
November 2000, pp. 78.) His oil exploration modeling entails
so-called elastic simulations that are about 250 times more computationally
intensive than standard acoustic (sound waves in air) simulations.
Elastic simulations provide better details of subsurface geology,
which is essential to oil exploration efforts, by depicting both
the S (shear) waves and the P (compressional) waves that travel
in water and earth. With computers such as TC2K, realistic three-dimensional
elastic simulations are now possible.
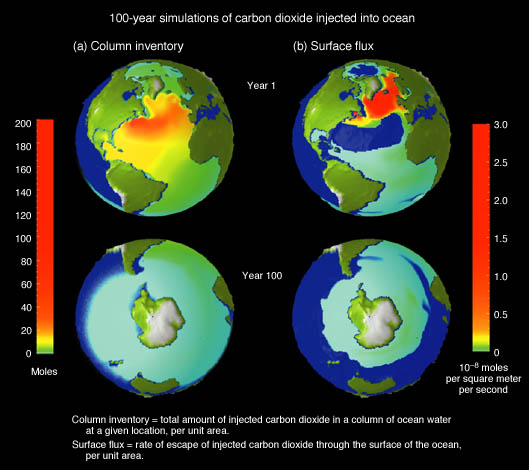 |
Multiprogrammatic
and Institutional Computing supercomputers are making it possible
to evaluate the potential effectiveness of injecting carbon
dioxide into the ocean to mitigate global warming. These 100-year
simulations depict what happens to carbon dioxide injected into
the ocean off New York City at a depth of 710 meters. The column
inventory, shown in the two spheres at the left, depicts the
carbon dioxide traveling a great distance in 100 years. The
surface flux, at right, shows that maximum escape of the injected
carbon dioxide can occur far from the injection site in areas
where there is vigorous overturning of the ocean, such as the
North Atlantic. |
Climate
Simulations Set the Pace
Livermore researchers in
atmospheric sciences were among the first to take advantage of M&ICs
new capabilities. Having performed parallel computing for 10 years,
they had already achieved some of the most advanced simulations
ever done. They had also made their simulation codes portable, that
is, easily adaptable to different computers. As a result, it did
not take long to adapt their codes to TC2K and ASCI Frost.
They put TC2K to the test
to study the effectiveness of ocean carbon sequestration, a proposed
approach for mitigating global warming. In one sequestration model,
the carbon dioxide generated by industrial operations would be injected
into the oceans instead of being emitted into the atmosphere. However,
some of the injected carbon dioxide would eventually leak into the
atmosphere, where it would contribute to climate change.
To evaluate this approach
to mitigating global warming, the Department of Energy formed a
Center for Research on Ocean Carbon Sequestration, located at Lawrence
Livermore and Lawrence Berkeley national laboratories. For one of
the centers studies, Ken Caldeira, codirector of the center,
along with colleagues Philip Duffy of Atmospheric Sciences and Mike
Wickett of the Center for Applied Scientific Computing, used TC2K
to evaluate the effectiveness of ocean carbon sequestration over
a period of 100 years. We want to know how much of the injected
carbon dioxide would leak out of the ocean and at what rate,
says Duffy. Their simulations showed that leakage into the atmosphere
is much less when carbon dioxide injection is done at greater depths.
The simulations also showed that maximum leakage may occur far from
the injection site in areas where there is vigorous overturning
of the ocean, such as in the North Atlantic. The simulations, the
highest resolution of their kind ever done, used up to a hundred
TC2K nodes and required a total of about 10,000 CPU hours.
Duffy and other atmospheric
researchers, together with collaborators from Livermores Center
for Applied Scientific Computing, have used TC2K and other DOE supercomputers
to perform the highest-resolution global climate simulations ever
done. Duffy notes that as the simulation resolution gets finer,
topographic features like mountains and valleys are represented
better; these features have a direct bearing on weather. The simulations
show that in some regions, maximum warming will occur in high-elevation
regions because of a snow-albedo feedback: warming causes reduced
snow cover, which in turn amplifies the warming by reflecting less
sunlight back into space.
The simulations were performed
in part on TC2K, in part at the National Energy Research Supercomputing
Center at Lawrence Berkeley, and in part on ASCI Frost. TC2K
has really allowed us to push the limits of model resolution,
says Duffy. Were doing things that no other researchers
can duplicate.
 |
Simulations
depict changes of surface air temperature between 2000 and 2100
in the
western U.S. The simulation on the left, produced on Livermores
ASCI Frost and TC2K supercomputers, has a 75-kilometer resolution.
The simulation on the right, produced on the National Energy
Research Supercomputing Center at Lawrence Berkeley National
Laboratory, has a 300-kilometer resolution. Its finer resolution
gives a more detailed prediction that should be more accurate. |
Unprecedented
Ozone Studies
Atmospheric scientists in
the Atmospheric Chemistry Group have used a hundred processors for
a total of about 400 hours in their studies of the atmosphere. They
have the only atmospheric chemistry model, IMPACT, that is capable
of simulating the chemical reactions occurring in both the troposphere
(the 10 kilometers of the atmosphere closest to Earth) and stratosphere.
Past studies modeled the troposphere and stratosphere independently
because of computational limitations. TC2K has enough computational
power to permit coupling the troposphere and stratosphere in an
atmospheric chemistry simulation capable of accurately predicting
ozone concentrations. The results showed that studying interactions
between these two regions of the atmosphere is important for understanding
global and regional ozone distributions.
Understanding the ozone distribution
throughout the atmosphere is crucial to the ability to predict not
only the possibility of future stratospheric ozone depletion but
also Earths radiation balance and the magnitude of global
warming. The scientists model included resolutions of 2 degrees
latitude by 2.5 degrees longitude, 46 levels of altitude from Earths
surface to 60 kilometers, tropospheric and stratospheric chemistry
and physics involving 100 chemical species and 300 chemical reactions,
and weather dynamics. Because our codes are CPU-intensive,
they do well on TC2K, says atmospheric scientist Doug Rotman.
The machine excels at big problems involving a lot of parameters.
He says that while one goal is to keep increasing the resolution
of the simulations, another goal is to include additional physics
to make simulations more realistic.
Engineers David Clague, Elizabeth
Wheeler, and Todd Weisgraber and University of California at Berkeley
student Gary Hon have been using TC2K to perform three-dimensional
simulations of both the stationary and mobile particles in portable
microfluidic devices. These devices are being designed by Livermore
researchers to automatically detect and identify viruses, bacteria,
and toxic chemicals. (See S&TR, November 1999, pp. 1016.)
The devices have channels from 20 to 200 micrometers deep and up
to a millimeter wide through which fluids travel. Because the channels
are so small, intermolecular forces, which are typically masked
in laboratory-scale instruments, affect the behavior of particles.
The simulations show how beads and macromolecules are affected by
each others electric fields as they travel through a channel.
 |
The
TC2K supercomputer permits simulations that for the first time
couple the troposphere and stratosphere in modeling the distribution
of ozone. Modeling results show that in various locations, ozone
in the lower stratosphere, which is typically in 100-parts-per-billion
concentrations, is transported to near-surface altitudes. |
 |
This
simulation using TC2K shows the influence of electric fields
on molecules traveling in narrow channels of portable microfluidic
devices. The devices are being designed to automatically detect
and identify viruses, bacteria, and toxic chemicals.
|
Initiative
a Big Success
McCoy is pleased
that M&IC computing resources have been so well received. One
sign of the initiatives success has been the growing competition
for the finite resources and the occasional wait of several days
to begin big simulation runs. The desktop diaspora is over,
says McCoy, and the result is unprecedented simulations and outstanding
science. We have achieved a balance in understanding what
can be best done on the desktop and what can be best done in the
experimental computational facility. We have developed close partnerships
with our science teams, and we are already planning the next steps.
McCoy adds it is the momentum
based on continual change that keeps me and the Scientific Computing
and Communications Department engaged and interested. That
interest, he says, is based in part on the newest generation of
simulations. They promise significant discoveries in science as
Livermore researchers continue to elevate simulation to a level
equal to that of theory and experiment.
—Arnie Heller
Key Words:
Accelerated Strategic Computing Initiative (ASCI), ASCI Blue Pacific,
ASCI Frost, ASCI White, atmospheric sciences, carbon sequestration,
Compass Cluster, elastic simulations, E3D, Institutional Computing
Executive Group, JEEP, microfluidic devices, Multiprogrammatic and
Institutional Computing Initiative, parallel computing, Scientific
Computing and Communications Department, Sunbert, supercomputers,
TeraCluster, TeraCluster2000 (TC2K), Terascale Simulation Facility.
For further
information contact Mike McCoy (925) 422-4021 (mccoy2@llnl.gov)
or Greg Tomaschke (925) 423-0561 (tomaschke1@llnl.gov).
About
the Scientist
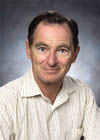 |
MIKE
McCOY,
deputy associate director of Scientific Computing and Communications
in Livermores Computation Directorate, received his A.B.
(1969) and Ph.D. (1975) in mathematics from the University of
California at Berkeley. He joined the Laboratory in 1975 as
a student employee. Upon completing his doctoral dissertation,
he became a staff scientist in the National Energy Research
Supercomputer Center, where he took responsibility for the development
of algorithms for plasma codes. He went on to become group leader
of the centers Massively Parallel Computing Group and
then its deputy director. In the latter role, he directed the
procurement of the 256-processor T3D computer, which was used
for unclassified science. Now, as deputy associate director
for Computation, he continues to support programs to advance
network communications and security and to foster the development
and integration of systems of powerful computers. He has worked
with Livermore science teams to establish institutional computing
to provide Laboratory scientists with access to powerful simulation
environments. This sharing of institutional resources is part
of his vision for enhancing the role of simulation in the scientific
triad of theory, experiment, and simulation. |
|